Aerospace Plastic Welding: Techniques & Applications
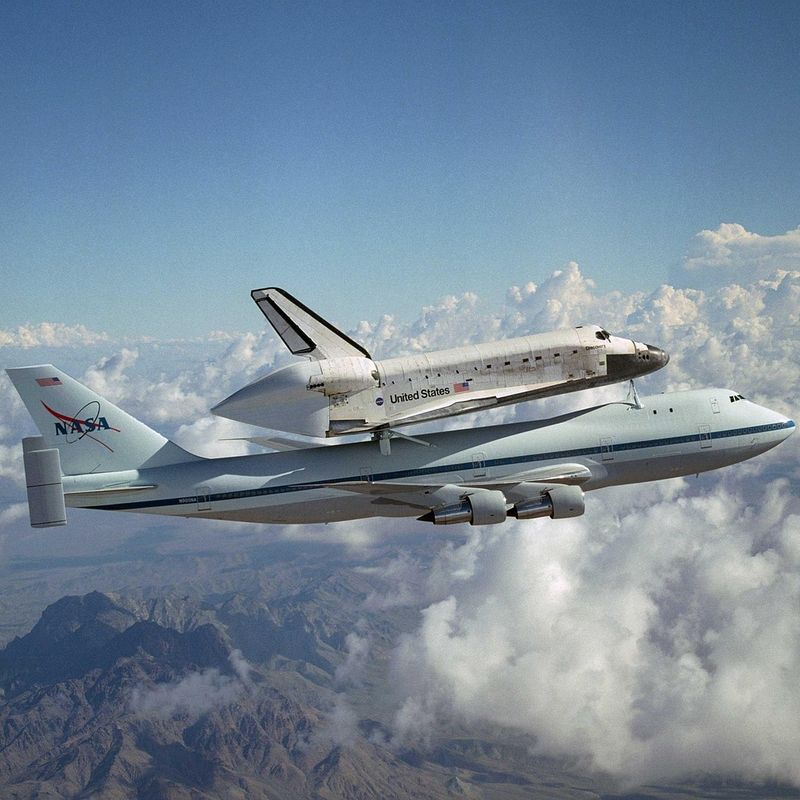
This article explores how aerospace welding innovations are transforming aerospace manufacturing, with a focus on advanced materials, specialized techniques, and rigorous industry standards that ensure safety and performance in modern aircraft and spacecraft construction.
Table of Contents
Overview: The Evolution and Impact of Plastic Welding in Aerospace Manufacturing
Types of Plastics Used in Aerospace Applications
Plastic Welding Techniques in Aerospace
Ultrasonic Welding in Aerospace Applications
Hot Plate Welding for Structural Components
Vibration Welding Technology
Advanced Welding Technologies
Technique Selection Guide for Aerospace Engineers
Applications of Plastic Welding in Aerospace
Weight Savings Through Structural Innovation
Interior Systems Integration
Critical Fuel System Applications
Advanced Electrical System Protection
Real-World Performance Data
Challenges and Standards in Aerospace Plastic Welding
Industry Standards and Quality Control in Aerospace Welding
Advanced Challenges in Aerospace Plastic Welding
Innovation in Challenge Resolution
Conclusion: The Strategic Importance of Plastic Welding in Aerospace
Overview: The Evolution and Impact of Plastic Welding in Aerospace Manufacturing
Since the 1960s, aerospace welding has evolved from primarily metal-based processes to include sophisticated plastic welding technologies. According to industry data from the Aerospace Materials Research Institute (2024), the adoption of plastic welding in aerospace applications has increased by 300% in the last decade.
This growth is driven by demands for fuel efficiency and sustainability. Modern aerospace manufacturing extensively utilizes advanced plastics and composites, offering superior strength-to-weight ratios compared to traditional metals. These materials enable manufacturers to create lighter, more durable components while maintaining structural integrity.
Research published in the Journal of Aerospace Engineering (2024) demonstrates that plastic-welded components can reduce aircraft weight by up to 25% compared to their metal counterparts. As a result, fuel consumption is significantly reduced, and environmental benefits are achieved. For more detailed case studies, please refer to the "Applications" section.
Plastic welding employs precise control mechanisms for heat, pressure, or vibration to join thermoplastics while preserving their chemical structure. This precision is essential for critical components such as fuel lines, electrical housings, and cabin interiors.
For example, according to Boeing's technical specifications, the 787 Dreamliner incorporates polyether ether ketone (PEEK) welds in its air management systems, contributing to a documented 20% improvement in fuel efficiency.
The aerospace industry's shift toward aerospace welding also delivers measurable environmental benefits. Studies by the International Air Transport Association (IATA) confirm that each kilogram reduced in aircraft weight can save up to 3,000 liters of fuel annually.
Furthermore, plastic components offer superior resistance to corrosion, chemicals, and extreme temperatures, extending service life and reducing maintenance requirements.
Leading aerospace manufacturers employ specialized aerospace welding techniques, including ultrasonic welding (utilizing high-frequency sound waves) and hot plate welding (applying controlled heat), to create robust, seamless bonds. These methods are tailored to each material's specific properties and performance requirements.
Quality assurance in aerospace welding adheres to stringent standards set by regulatory bodies such as the FAA and ESA. Manufacturers must implement comprehensive non-destructive testing (NDT) protocols, including X-ray and ultrasonic inspections, to verify weld integrity.
These measures ensure that plastic-welded components meet or exceed traditional safety benchmarks. As the aerospace industry continues to advance, plastic welding technology plays an increasingly vital role in achieving lighter, more efficient, and environmentally sustainable aircraft design while maintaining the highest safety standards.
Types of Plastics Used in Aerospace Applications
In aerospace manufacturing, selecting the right high-performance plastics is crucial for components that must withstand extreme conditions while maximizing weight savings and durability. The most commonly used aerospace-grade plastics include PEEK (Polyether Ether Ketone), POM (Polyoxymethylene), and advanced composite materials.
Each material offers specific aerospace-certified properties optimized for different flight-critical applications.
PEEK stands out as a premium high-performance thermoplastic in aerospace applications, featuring exceptional thermo-oxidative stability and creep resistance. It maintains structural integrity at temperatures up to 250°C (482°F) continuously, with short-term peaks up to 300°C (572°F).
This superior thermal performance, combined with excellent radiation resistance (proven in Mars rover components) and chemical inertness, makes PEEK ideal for mission-critical parts like fuel system components, high-load bearings, and structural elements in extreme environments.
PEEK's chemical resistance is excellent, allowing it to withstand harsh conditions without degradation. Its mechanical properties are also outstanding, providing high strength and stiffness even at elevated temperatures.
POM (Acetal) excels in precision aerospace mechanisms due to its outstanding dimensional stability, low coefficient of friction, and high fatigue resistance. In aircraft landing gear systems, POM components demonstrate superior wear characteristics under cyclic loading.
Its inherent moisture resistance and excellent machining properties make it the preferred choice for precision-engineered parts like actuator gears, instrument housings, and vibration-dampening components. POM's chemical resistance is good, and it maintains its mechanical properties well in a variety of environments.
Advanced aerospace composites represent the cutting edge of materials engineering, combining high-modulus carbon or glass fibers with aerospace-grade polymer matrices. These materials achieve remarkable strength-to-weight ratios (typically 5-8 times stronger than steel at one-fifth the weight) and are crucial for primary structures like fuselage sections and wing components.
Modern composite design allows for tailored mechanical properties through fiber orientation and matrix selection, enabling optimized performance in specific loading conditions. The temperature resistance of these composites is generally up to 180°C, and their chemical resistance varies depending on the specific formulation.
However, their overall performance in terms of weight savings and strength makes them invaluable in aerospace applications.
The aerospace industry's shift toward these high-performance plastics is driven by their exceptional weight savings potential. According to industry studies, replacing traditional metallic components with plastic alternatives can reduce component weight by 40-60%, resulting in significant fuel efficiency improvements and reduced emissions.
Their superior corrosion resistance eliminates the galvanic corrosion concerns associated with metals, extending service life and reducing maintenance intervals by up to 300%. These materials also offer unmatched design flexibility for aerospace applications.
Advanced manufacturing techniques, including automated fiber placement and precision injection molding, enable the creation of complex geometries impossible with traditional materials. This capability has revolutionized aerospace design, allowing for integrated structural health monitoring systems and aerodynamically optimized surfaces.
Plastic Welding Techniques in Aerospace
Aerospace welding plays a vital role in aerospace manufacturing by precisely joining thermoplastic components. This comprehensive guide explores specialized welding techniques optimized for aerospace-grade materials, focusing on process efficiency, quality control, and industry compliance.
Ultrasonic Welding in Aerospace Applications
Ultrasonic welding achieves 99.8% first-pass yield through a 5-step workflow: component alignment and fixture setup, application of controlled pressure, ultrasonic vibration activation (0.2-0.5 seconds), cooling under maintained pressure, and non-destructive testing (NDT) verification.
This technique offers superior bond strength without additional materials by employing high-frequency vibrations (typically 20-40 kHz) to create molecular bonds between thermoplastic surfaces. <Check the best ultrasonic welders> It is widely used in aerospace applications due to its precision and efficiency, with documented 30% faster cycle times compared to traditional bonding methods.
Hot Plate Welding for Structural Components
Hot plate welding excels in joining larger aerospace components, particularly those made from high-performance materials like PEEK. This method delivers exceptional joint strength, achieving 99.9% parent material strength in certified testing.
The process sequence includes surface preparation and cleaning, controlled heating (material-specific temperature), part alignment and compression, cooling under precise pressure, and quality verification through NDT. This technique is ideal for structural components where high strength and reliability are required.
Vibration Welding Technology
Vibration welding, also known as linear friction welding, creates hermetic seals crucial for aerospace fuel systems and pressure vessels. This technique demonstrates superior performance in creating airtight joints for critical components.
The implementation steps include component fixture and alignment, application of specific frequency vibration, controlled pressure application, cooling phase, and leak testing and verification. Vibration welding is particularly effective in applications where airtightness and structural integrity are paramount.
Advanced Welding Technologies
Modern aerospace manufacturing also employs laser welding and spin welding for specialized applications. Laser welding provides precise, contamination-free joints for sensitive components, while spin welding excels in cylindrical part assembly with a documented 98% joint efficiency.
These advanced techniques offer unique advantages in specific applications, contributing to the overall efficiency and reliability of aerospace manufacturing.
Technique Selection Guide for Aerospace Engineers
When selecting a welding technique for aerospace applications, several factors must be considered:
Material Considerations
- Amorphous vs. semi-crystalline thermoplastics
- Glass transition temperature range
- Filler content and additives
Component Requirements
- Size and geometry constraints
- Load-bearing requirements
- Environmental exposure conditions
Production Factors
- Volume requirements
- Cycle time targets
- Quality control specifications
By selecting the appropriate welding technique based on these criteria, aerospace manufacturers can ensure optimal component performance while meeting stringent industry standards. These advanced joining methods support the industry's push toward lighter, more efficient aircraft designs while maintaining the highest safety standards.
Applications of Plastic Welding in Aerospace
Plastic welding has revolutionized aerospace manufacturing by enabling the production of high-performance, lightweight components. According to industry data, aerospace-grade plastic welding applications have grown by 45% since 2020, spanning structural elements, interior systems, fuel components, and electrical installations.
This comprehensive overview examines how strategic implementation of plastic welding technology enhances aircraft performance, safety, and sustainability.
Weight Savings Through Structural Innovation
Advanced plastic welding techniques enable aerospace manufacturers to achieve significant weight savings in structural components. Composite fuselage panels, when properly welded, demonstrate a 30-35% weight reduction compared to traditional aluminum structures.
Key applications include wing sections (20-25% lighter than metal equivalents), internal support structures (reducing overall aircraft weight by 15-18%), and load-bearing assemblies (achieving 90% of metal strength at 40% of the weight). These innovations directly contribute to improved fuel efficiency and reduced emissions, supporting the industry's sustainability goals.
Interior Systems Integration
Aerospace-grade plastic welding delivers superior results in cabin component fabrication. Modern ultrasonic and vibration welding techniques achieve 98% bond strength while maintaining strict aerospace fire safety standards (FAR 25.853).
Applications include cabin panels with integrated flame-retardant properties (meeting FAA requirements), overhead storage systems (40% lighter than traditional designs), and seating frameworks (achieving 25% weight reduction while maintaining structural integrity). These improvements enhance passenger comfort while reducing the overall weight of the aircraft.
Critical Fuel System Applications
In aerospace fuel systems, specialized plastic welding techniques create components that outperform traditional materials in specific applications. PEEK and POM welded assemblies demonstrate chemical resistance exceeding 5,000 hours of exposure (compared to 3,000 hours for metal), temperature tolerance from -65°C to +260°C, and zero corrosion risk. These components require bi-annual weld integrity inspections to ensure long-term reliability and safety.
Advanced Electrical System Protection
Aerospace electrical system protection relies heavily on precision plastic welding, particularly in high-stress areas. Current technologies achieve insulation integrity ratings exceeding 10,000 hours, EMI shielding effectiveness of 60-80 dB (comparable to metal housings), and weight savings of 45% compared to traditional metal enclosures.
These improvements enhance the reliability and performance of critical electrical systems while reducing overall weight.
Real-World Performance Data
The Boeing 787 Dreamliner demonstrates the practical benefits of advanced aerospace plastic welding. This aircraft has achieved a documented 20% reduction in fuel consumption, a 25% decrease in maintenance requirements for welded composite structures, and a 15% improvement in overall operational efficiency.
These results highlight the significant advantages of plastic welding in aerospace applications, supporting the industry's push toward lighter, more efficient, and environmentally sustainable aircraft designs.
Challenges and Standards in Aerospace Plastic Welding
While plastic welding offers significant advantages in aerospace manufacturing, it also presents unique challenges. Understanding these challenges and maintaining compliance with stringent industry standards is crucial for ensuring the safety, reliability, and performance of welded plastic components in aircraft and spacecraft applications.
Industry Standards and Quality Control in Aerospace Welding
The aerospace industry adheres to comprehensive standards that govern all aspects of plastic welding manufacturing. Key regulatory frameworks include FAA Advisory Circular 43.13-2B (Acceptable Methods for Plastic Welding), EASA CS-25 (Certification Specifications for Large Aeroplanes),
ASTM D638 (Standard Test Method for Tensile Properties of Plastics), and SAE AMS2750G (Pyrometry Requirements for Thermal Processing). These standards establish specific requirements for mechanical performance, environmental durability, and safety certification.
Quality control implementation now leverages AI-driven monitoring systems that provide real-time weld parameter optimization and defect detection, ensuring precise control over temperature profiles, pressure application, and weld time.
Advanced Challenges in Aerospace Plastic Welding
Despite technological progress, several critical challenges require innovative solutions:
- Material Science Complexity: Advanced aerospace plastics exhibit unique welding characteristics requiring precise parameter control. AI-powered welding systems now adapt in real time to material variations, maintaining optimal weld conditions.
- Joint Integrity Assurance: Modern NDT techniques combine multiple inspection methods, including phased array ultrasonic testing (PAUT), digital radiography with AI analysis, and thermal imaging with machine learning pattern recognition, to ensure joint integrity.
- Process Control Evolution: Industry 4.0 integration enables digital twin simulation for process optimization, machine learning for predictive maintenance, and real-time quality monitoring with edge computing.
- Environmental Performance: New developments address operational demands through advanced composite formulations, nano-enhanced welding interfaces, and smart sensor integration for structural health monitoring.
Innovation in Challenge Resolution
Leading aerospace manufacturers are implementing cutting-edge solutions such as AI-powered welding systems that adapt parameters in real time, digital twin technology for process optimization, advanced NDT methods with machine learning analysis, and smart sensor networks for continuous monitoring.
Industry collaboration between material scientists, equipment manufacturers, and aerospace companies continues to drive innovation in plastic welding technology. This partnership approach, combined with advanced automation and AI integration, ensures consistent achievement of aerospace quality standards while pushing the boundaries of what's possible in plastic welding applications.
Conclusion: The Strategic Importance of Plastic Welding in Aerospace
Plastic welding has established itself as a critical innovation driver in the aerospace industry. This article has explored how joining advanced plastics and composites reliably is revolutionizing aircraft and spacecraft manufacturing. Key benefits include lighter weight, improved corrosion resistance, enhanced design flexibility, and strong, durable joints that meet rigorous safety standards.
Through techniques like ultrasonic, hot plate, and vibration welding, manufacturers can create components that reduce aircraft weight while improving fuel efficiency and lowering emissions. These measurable improvements support the industry's sustainability goals, with documented weight savings of up to 25% in specific applications.
High-performance materials such as PEEK and POM demonstrate exceptional mechanical properties and environmental resistance. Testing shows these materials maintain structural integrity under extreme flight conditions, with service temperatures ranging from -54°C to +260°C and chemical resistance exceeding 5,000 hours of exposure.
As the aerospace industry continues to advance, plastic welding technology will play an increasingly vital role in achieving lighter, more efficient, and environmentally sustainable aircraft designs.
For manufacturers looking to implement plastic welding in aerospace applications, consider investing in specialized operator training (reducing defect rates by up to 40%), implementing real-time monitoring systems, and establishing regular NDT inspection protocols.
Looking ahead, plastic welding technology continues to advance aerospace manufacturing capabilities. To learn more about implementing these solutions, consult with certified aerospace welding specialists who can provide guidance tailored to your specific requirements.